A mere quarter century ago, the only planets that we knew about orbited our Sun. Astronomers didn’t know whether our solar system—eight planets, plus assorted moons, asteroids, and comets—was an analogue for other planetary systems and had no direct evidence that other planetary systems even existed at all.

Now, thanks to the recent findings of NASA’s Kepler space telescope (Figure 1), our understanding of our galactic neighborhood has drastically changed. We know that we live in a galaxy full of planets that lie outside of our solar system.
These bodies, referred to as extrasolar planets, or exoplanets, include many kinds of objects and showcase a level of diversity that took astronomers by surprise. Extrasolar planets include gas giants and rocky worlds so close to their stars that they whip around them in less than one Earth week and planets only a few times as massive as Earth that have voluminous low-density atmospheres, like shrunken versions of Uranus or Neptune.
This diversity shook up scientists’ ideas about how planets form and what a “normal” planetary system looks like. Now, 20 years to the month after scientists’ first discovery of a planet around a star other than our Sun, we can take stock of how Kepler has revolutionized our understanding of worlds beyond our solar system.
The Hunt for Earth-Like Worlds
The search for planets outside of our solar system dates back more than 150 years, but early claims of discovery turned out to be erroneous. The first exoplanets discovered that have withstood the test of time are two small planets orbiting a pulsar [Wolszczan and Frail, 1992], but pulsars are stellar remnants rather than true stars, and few other planets have been discovered in orbit about them.
The floodgates opened 20 years ago this month with the discovery of 51 Pegasi b [Mayor and Queloz, 1995], a planet about half as massive as Jupiter whipping around a star not unlike our Sun, with an orbital period of only 4.23 days. Hundreds of planets have since been found from the ground, but all are much larger than Earth and/or orbit very close to their stars and are consequently very hot.
To find smaller worlds, NASA launched the 0.95-meter-aperture Kepler space telescope into an orbit around the Sun in 2009 [Borucki et al., 2010; Koch et al., 2010]. Above Earth’s atmosphere and away from the glare and temperature variations of low Earth orbit, Kepler simultaneously monitored more than 150,000 stars, measuring their brightness every 30 minutes.
Kepler’s mission centered on searching for periodic dips caused by planets crossing in front of their host stars as they orbited—events called transits. The spacecraft stared at the same patch of sky for 4 years, until the failure of two reaction wheels—the gyroscope-like flywheels that point and stabilize the craft—which ended the main mission in May 2013.
Scanning for transits requires high-precision photometry. Although the transit of a Jupiter-size planet across the disk of a Sun-like star blocks 1% of the stellar flux and can readily be identified using a ground-based telescope, an Earth-size transiting planet dims the same star by less than one hundredth of a percent.

Kepler’s stability and custom-designed electronics allowed it to detect patterns of dips in brightness suggestive of more than 4600 planet candidates (Figure 2). Statistical analysis indicates that the vast majority of these signals represent true exoplanets.
In some respects, many of these systems look like miniature versions of our own solar system. Most of the planet candidates found by Kepler have years that are shorter than a few Earth months and sizes in between those of Earth and Neptune, a size that our solar system lacks. In addition, their orbits can be much more tightly spaced. More than one third orbit in systems where multiple transiting planets have been found, and Kepler data tell us that systems containing multiple planets on closely spaced orbits are quite common.
A Bounty of Planets
Individual planets and planetary systems can be exciting and illuminating discoveries [Lissauer et al., 2014]. However, Kepler is primarily a statistical mission, designed to determine the abundance of planets as small as Earth that orbit within their star’s habitable zones—the distance where planets with an atmosphere similar to Earth’s receive the right amount of stellar radiation to maintain reservoirs of liquid water on their surfaces.
Kepler measures the sizes of planets when they flit in front of their host star: The bigger the dip in brightness is, the larger the size of the planet is relative to its star. Astronomers determine the size and density of the host star itself by combining ground-based observations with stellar models. In some cases, Kepler data can further refine these estimates on the basis of tiny variations in brightness caused by the stars’ internal vibrations or by the shapes and lengths of the transits themselves.
Planets smaller than Neptune are far more common than giant planets.
This ability to size up planets has led to the realization that planets smaller than Neptune (which is 4 times as large as Earth) are far more common than giant planets. Although the number changes from catalog to catalog, more than half of the planet candidates found by Kepler are 1–3 times the size of Earth and take less then 100 days to orbit their stars [Batalha, 2014]. The number of planets drops off gradually for periods below 10 days, but planets with periods as short as about 8 hours have been found.
What Are Exoplanets Made of?
To find out what a planet is made of—and whether it is rocky, Earth-like, and potentially capable of supporting life—astronomers need to determine not just the size of a planet but also its mass. Traditionally, many of these mass measurements have come from the Doppler technique, which typically uses large ground-based telescopes to measure how the light of a host star shifts to redder and bluer wavelengths as it rocks back and forth in response to the gravitational tugs of the planet. This works best on planets with very short period orbits around relatively bright stars [Marcy et al., 2014].
Kepler isn’t able to directly measure masses (except for a few massive planets on very short period orbits that tidally distort their star to a measurable degree). However, astronomers can sometimes use Kepler data to indirectly determine the masses of planets in multiplanet systems by studying how their gravitational tugs pull on each other, which very slightly disturbs their orbits.
Such perturbations cause orbital paths to deviate from purely mathematical ellipses, speeding them up or slowing them down and interrupting the otherwise clockwork timing of their transits. The masses of dozens of Kepler planets, including some not much larger than Earth and others with orbital periods of up to a few months, have been measured by studying the transit timing variations (TTVs) produced by these perturbations.

The difference between planet transit timing of single- and multiple-planet systems. In tightly packed planetary systems, the gravitational pull of the planets among themselves causes one planet to accelerate and another planet to decelerate along its orbit. The acceleration causes the orbital period of each planet to change. Kepler detects this effect by measuring the change known as transit timing variations. Credit: NASA Ames/Kepler mission, Standard YouTube License

These measurements have revealed a class of planets astronomers didn’t know existed: low-mass, gas-rich worlds. In fact, most planets with radii between 2 and 8 times that of Earth and orbital periods less than 100 days have quite low density, indicating that most of the planets’ volume is filled with hydrogen and helium gas. However, using mass measurements, scientists know that heavier elements must comprise the vast majority of the masses of most of these large and light worlds.
There appears to be a fuzzy boundary between Earth-like rocky worlds with a thin atmosphere and these mini-Saturns with large, fluffy layers of gas. The data currently suggest that the upper end of the boundary region between rocky and other planets is at about 1.6 times the size of Earth (Figure 3). However, mass data remain sparse, are measurable only for planets around very bright stars, and suffer from detectability biases, so it remains unclear where the lower end of this boundary region lies.
Multiplanetary Systems
Kepler was designed to search for small planets in the habitable zone, but its ultraprecise, long-duration photometry is also ideal for finding systems with multiple transiting planets (multis). Kepler has discovered more than 690 candidate multis, almost all of which represent true multiple-planet systems.
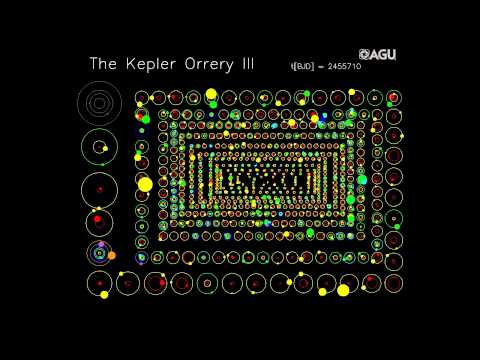
The multi-transiting planetary systems discovered by Kepler up to November 2013. The colors show the order of the orbits from the star; the most colorful is the 7-planet system Kepler-90. The orbits share a relative scale; radii of the planets share a different scale. The terrestrial planets of the solar system are shown in gray. Credit: Daniel Fabrycky, University of Chicago
The multitransiting systems observed by Kepler provide a large and very rich data set of short-period planets (with orbits cycling every few days to several months, in Earth terms) that can be used to powerfully test theoretical predictions of the formation and evolution of planetary systems. For one, the sheer number of Kepler multis implies that such systems with multiple close-in planets are common, with about 5% of stars having two or more planets that are at least 1.5 times as large as Earth and that complete full orbits in less than 100 Earth days.
Candidate systems with two or more transiting planets seem to orbit close to the same plane [Fabrycky et al., 2014], suggesting mutual inclinations similar to our solar system. Orbits are nearly circular, with typical eccentricities of only a few percent. This ellipticity is similar to our solar system but small compared to those of most giant exoplanets with orbital periods of more than 10 days.
How Common Are Planets Like Earth?
Although Kepler has discovered planets smaller than Earth, these are hot worlds orbiting close to their stars or orbit stars much smaller than our Sun. Probably the best Earth analogue to date is Kepler-62f, which is 40% larger than Earth and therefore has a fairly good chance of being rocky. The exoplanet orbits a star about 30% smaller than the Sun at the right distance to have liquid water on its surface if it has an Earth-like atmosphere. Another possible Earth analogue is Kepler-186f, intermediate in size between Earth and Kepler-62f. This orb is likely to be rocky, but it orbits a much smaller star and thus is likely to be in a tougher environment for life as we know it to flourish.
Scientists expect to find hundreds if not thousands of additional Kepler planet candidates.
As scientists continue to analyze the Kepler data, they expect to find hundreds if not thousands of additional Kepler planet candidates. In addition, better estimates of stellar parameters will lead to more accurate values for planetary sizes. Many more candidates will be verified as planets, and some of the false positives will be removed from the candidate pool. Many of the planets will be characterized better through future observations and analysis of TTVs.
As the numbers improve, scientists will seek to better quantify the fraction of stars hosting Earth analogues. This is of great interest to astrobiologists—it’s an important step in determining how common the conditions for life are in the galaxy.
Prior to Kepler, the best estimates on the number of Earth analogues were based not on data but on theoretical models of planet formation. So far, Kepler has provided very good estimates of the abundance of Earth-sized planets only for those near their stars, which are easier to detect. Extrapolating these numbers suggests that Earth analogues are fairly common, albeit far from ubiquitous.
As data analysis proceeds, however, scientists will find more longer-period planet candidates, and good figures for the abundance of Earth-sized planets will probably be available for those with orbiting periods out to 100 days. At periods of 1 year, good abundances will probably be available for planets twice the size of Earth.
These data will allow much better estimates of the number of Earth analogues, requiring less extrapolation. Some extrapolation will still be necessary and will extend in two directions: upward in period for Earth-size planets and downward in size for planets with orbital periods similar to that of Earth. This will provide a consistency check on results.
What’s Next for Exoplanet Studies?
Although the hobbled Kepler spacecraft cannot observe its original star field any longer, its engineers were able to reconfigure the craft for a new mission, dubbed K2, to continue its search for other worlds. With only two good reaction wheels, the craft can maintain the necessary stability along only two axes. To remain stable enough to take good data, Kepler must point nearly parallel to its orbital path around the Sun so that sunlight falls on it evenly and the resulting pressure doesn’t send the spacecraft into a spin.
K2 can study a specific patch of sky for up to 83 days—at which point the spacecraft must rotate to prevent sunlight from entering the telescope. Since Kepler typically needs to see two or three transits before declaring a signal to be a potential planet, it is primarily discovering planets with orbital periods of less than 30 days.
The European Space Agency’s Gaia spacecraft, launched in 2013, is also aiding exoplanet scientists by helping to determine the distances to Kepler planet–hosting stars very accurately, enabling better estimates of their sizes and therefore of the sizes of their planets. Gaia will also discover new planets, primarily massive ones with orbital periods of 1 to a few years around nearby stars, from observing the wobble that these planets induce in the positions of their stars on the sky.
Kepler will soon have a true spiritual successor: The Transiting Exoplanet Survey Satellite, or TESS, a NASA mission scheduled to launch in 2017, will scan the entire sky for transiting planets around the nearest and brightest stars. Their brightness means that the planets TESS discovers will be easier targets for follow-up observations from the ground and NASA’s James Webb Space Telescope (to launch in 2018).
These findings will continue to improve our understanding of how rare or ubiquitous planets like Earth are in our galaxy.
TESS will observe Kepler planets during a portion of its mission, which will allow it to collect more observations of TTVs for those targets. This will be very useful; having TTVs that span a large range of time greatly improves the accuracy of the measurements of the masses and orbital characteristics of the planets. Planetary Transits and Oscillations of Stars (PLATO), a European mission scheduled for launch by 2024, will also take additional TTV measurements for Kepler targets, as will ground-based telescopes.
These findings will continue to improve our understanding of the masses and radii of rocky exoplanets—and ultimately how rare or ubiquitous planets like Earth are in our galaxy.
References
Batalha, N. M. (2014), Exploring exoplanet populations with NASA’s Kepler Mission, Proc. Natl. Acad. Sci. U. S. A., 111, 12,647–12,654.
Borucki, W. J., et al. (2010), Kepler Planet-Detection Mission: Introduction and first results, Science, 327, 977–980.
Fabrycky, D. C., et al. (2014), Architecture of Kepler’s multi-transiting systems: II. New investigations with twice as many candidates, Astrophys. J., 790, 146.
Koch, D. G., et al. (2010), Kepler mission design, realized photometric performance, and early science, Astrophys. J., 713, L79–L86.
Lissauer, J. J., R. I. Dawson, and S. Tremaine (2014), Advances in exoplanet science from Kepler, Nature, 513, 336–344.
Marcy, G. W., et al. (2014), Masses, radii, and orbits of small Kepler planets: The transition from gaseous to rocky planets, Astrophys. J. Suppl., 210, 20.
Mayor, M., and D. Queloz (1995), A Jupiter-mass companion to a solar-type star, Nature, 378, 355–359.
Wolszczan, A., and D. A. Frail (1992), A planetary system around the millisecond pulsar PSR1257+12, Nature, 355, 145–147.
Author Information
Jack J. Lissauer, Space Science and Astrobiology Division, NASA Ames Research Center, Moffett Field, Calif.; email: jack.lissauer@nasa.gov
Citation: Lissauer, J. J. (2015), Kepler: A giant leap for exoplanet studies, Eos, 96, doi:10.1029/2015EO035131. Published on 15 September 2015.
Text not subject to copyright.
Except where otherwise noted, images are subject to copyright. Any reuse without express permission from the copyright owner is prohibited.