Hydrothermal vents act like pores in Earth’s skin, providing portals for the exchange of heat and chemicals between Earth’s interior and the overlying ocean. These vents play a major role in the Earth-ocean system, coupling submarine magmatic, tectonic, biological, and hydrothermal processes.
COVIS recorded a 4-year data set while it was connected to the world’s first high-power, high-bandwidth cabled seafloor observatory.
Vent systems and their interactions have been a subject of intense study over the past few decades [Kelley et al., 2014]. Heat flux, the amount of heat discharged from a vent site over a given time span, is one of the most important field measurements for understanding these interactions [Rona et al., 2015]. Despite the importance of quantifying the amount of heat discharged from a hydrothermal system over time and space, this task has proven challenging for scientists.
We have tackled this challenge using an unprecedented long-term acoustic data set, spanning more than 4 years, that captures hydrothermal venting off the coast of the Pacific Northwest region of North America. The Cabled Observatory Vent Imaging Sonar (COVIS) recorded this data set while it was connected to the world’s first high-power, high-bandwidth cabled seafloor observatory: the North East Pacific Time-series Underwater Networked Experiments (NEPTUNE) observatory. Ocean Networks Canada (ONC) operates NEPTUNE at the Endeavour segment of the Juan de Fuca Ridge, just off the coast of British Columbia.

Going Beyond Snapshots and Keyhole Views
Almost all heat flux measurements made to date are either one-time snapshots or annually repeated samples. Thus, they have limited application for studying the gradual evolution of seafloor venting at the finer timescales. Furthermore, many of these measurements have limited spatial coverage and resolution. Extrapolating those spot measurements over larger areas like a vent field can produce results with substantial uncertainty [Rona et al., 2015].

New instruments like our COVIS (Figure 1), connected to cabled seafloor observatories, show considerable potential for quantitative monitoring of heat and vent fluid fluxes of discharge over significant spatial areas (~100 × 100 meters) of seafloor hydrothermal vent sites.
The acoustic data from COVIS yield 3-D images of focused-flow areas, including plumes rising tens of meters from black smoker vents on a sulfide structure named Grotto. COVIS also produces 2-D maps of the distribution of diffuse flow venting in the surrounding areas where optically transparent hydrothermal fluids discharge at relatively low temperatures.
More important, further analysis of the acoustic data yields time series measurements of the flow rate, volume flux, and, subsequently, the heat flux of hydrothermal venting. These measurements are essential for studying how a hydrothermal system changes over time and how it interacts with geological, oceanic, and biological processes.
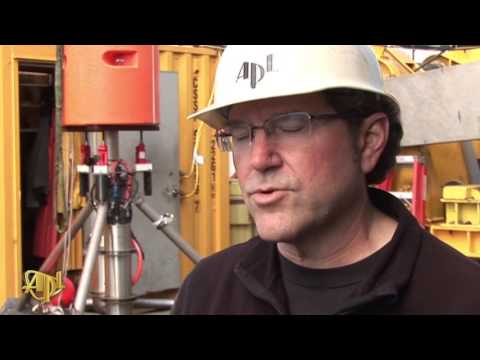
What Is COVIS?
COVIS was specifically designed to acquire water column multibeam backscatter data for imaging hydrothermal plumes and measuring their vertical velocity and heat flux and to use acoustic backscatter from the seafloor to detect and monitor diffuse hydrothermal discharge. COVIS uses a multibeam sonar with three-axis rotation (Figure 2) mounted on a 4.2-meter tower. The electronics and data-handling hardware are contained in a pressure vessel near the base of the tower.

COVIS evolved from an initial theoretical study to determine whether black smoker plumes could be imaged acoustically in the 1980s. In the 1990s and early 2000s, researchers used acoustic backscattering to image plumes using various sonars mounted on human-occupied and remotely operated vehicles (ROVs). Later, scientists extended acoustic methods to image and measure both focused and diffuse flow [Bemis et al., 2015].
In 2000, a sonar mounted on ROV Jason achieved a milestone along this development timeline. Jason’s 23-hour series of acoustic images of plumes and diffuse flow at the Grotto vent cluster in the Main Endeavour Field marks the transition from snapshots to time series observations of hydrothermal flow [Rona et al., 2006]. Even that short time series shows the plume alternately bending northeast and southwest, with corresponding variations in mixing with ambient seawater driven by tidal currents.
Most recently, COVIS was installed and connected to ONC’s NEPTUNE observatory at the Grotto vent cluster in the Main Endeavour Field on the Juan de Fuca Ridge. COVIS operated at Grotto (with a few interruptions for cable repairs and network outages) from 29 September 2010 to 10 September 2015, collecting an unprecedented time series of hydrothermal flow. The current COVIS database consists of about 4 years of plume images, Doppler vertical flow, volume flux, heat flux, and diffuse discharge maps at various time intervals (Figures 3 and 4); these data can be accessed at the Ocean Networks Canada Oceans 2.0 website.

From Sonar Echoes to Heat Flux
The water column imaging capabilities of COVIS build on standard backscatter-based imaging. We increase the signal-to-noise ratio by replacing the magnitude of backscatter from one ping with the magnitude of the waveform difference in backscatter between successive pings. COVIS’s imaging is sensitive primarily to rapid, turbulent temperature fluctuations in high-temperature plumes and secondarily to particulates [Xu et al., 2017].
These observations capture the response of hydrothermal systems to volcanic eruptions, earthquakes, ocean tides, and even surface winds.
In an alternative mode, COVIS data are processed for Doppler phase shifts to estimate line-of-sight velocities of reflected sound waves. These velocities can then be geometrically converted to yield estimates of plume vertical velocities, which are then used to estimate the volume of material flowing from the vent. We then use the theoretical dependence of heat flux on the vertical variation of volume flux to calculate the heat flux.
COVIS demonstrated the ability to monitor hydrothermal venting by producing a 4-year record with 3-hour temporal resolution of heat flux from a focused vent cluster (Figure 4) [Xu et al., 2014]. It has also monitored the areal extent of diffuse venting with submeter resolution over a 30 × 30 meter region [Rona et al., 2015] at the Grotto vent (Figure 3).
This combination of spatial and temporal coverage and resolution is unmatched by any previous measurement. These observations demonstrate the ability to capture the response of hydrothermal systems to such external forcings as volcanic eruptions, earthquakes, ocean tides, and even surface winds [Xu et al., 2013, 2014].
What Has COVIS Shown Us?
The heat flux time series shown in Figure 4 implies that the heat source driving the venting at Grotto remained relatively steady over the 26-month period. Comparison with seismic measurements taken over the same time period indicates that this period of steady hydrothermal heat output coincides with a period of reduced local seismicity.

Further comparison with historical heat flux and seismic measurements suggests that the evolution of hydrothermal heat output from Grotto since 1988 correlates with the rate of local seismicity. Short episodes of increased heat flux followed pronounced seismic activities, and longer periods of reduced steady heat flux occurred during periods of quiescent seismicity [Xu et al., 2014].
To detect diffuse flow venting, COVIS detects the phase changes caused by sound travel time variations, which, in turn, are caused by variations in the water temperature between COVIS and the diffuse flow–covered seafloor. We expect that the backscatter from the seafloor remains constant, so those phase changes indicate the presence of diffuse flow and can be used to create 2-D maps of this flow (Figure 3d).
The areal extent of diffuse flow shown in those maps, combined with in situ temperature and flow rate measurements, yields estimates of about 33 megawatts of total heat flux from diffuse venting at Grotto [Rona et al., 2015]. In addition, the 2-D maps created in 3-hour intervals reveal the temporal variations of the diffuse flow area at Grotto, which can be further used to study the influences of bottom currents and seafloor tidal pressure on diffuse flow venting.
Acoustic Imaging: A Promising New Tool
COVIS has demonstrated the viability of acoustic imaging for measuring heat flux from hydrothermal vent clusters and their surroundings.
COVIS has collected an unprecedented data set capturing the heat flux of high-temperature venting and the local distribution of diffuse discharge over a 4-year period at the Grotto sulfide vent cluster. COVIS has demonstrated the viability of acoustic imaging for the quantitative measurement of heat flux from high-temperature hydrothermal venting across a vent cluster and the qualitative capture of diffuse venting in the surroundings.
New developments in acoustic methods promise to increase quantitative applications to the heat flux of diffuse venting and to broaden the application of acoustic imaging to alternate platforms. Our ongoing work focuses on using the acoustic signal to quantify the intensity of the diffuse venting in the areas where we mapped the distribution of this activity. Three-dimensional imaging capabilities are potentially valuable for interpreting the sources of anomalies in sensor data collected along a vehicle track. Thus, a future challenge is to transfer these techniques from a stationary platform to a moving vehicle.
References
Bemis, K. G., et al. (2015), The path to COVIS: A review of acoustic imaging of hydrothermal flow regimes, Deep Sea Res., Part II, 121, 159–176, https://doi.org/10.1016/j.dsr2.2015.06.002.
Kelley, D. S., J. R. Delaney, and S. K. Juniper (2014), Establishing a new era of submarine volcanic observatories: Cabling Axial Seamount and the Endeavour segment of the Juan de Fuca Ridge, Mar. Geol., 352, 426–450, https://doi.org/10.1016/j.margeo.2014.03.010.
Rona, P. A., et al. (2006), Entrainment and bending in a major hydrothermal plume, Main Endeavour Field, Juan de Fuca Ridge, Geophys. Res. Lett., 33, L19313, https://doi.org/10.1029/2006GL027211.
Rona, P. A., et al. (2015), Estimations of heat transfer from Grotto’s North Tower: A NEPTUNE Observatory case study, Deep Sea Res., Part II, 121, 95–111, https://doi.org/10.1016/j.dsr2.2015.05.010.
Xu, G., et al. (2013), Observations of the volume flux of a seafloor hydrothermal plume using an acoustic imaging sonar, Geochem. Geophys. Geosyst., 14(7), 2369–2382, https://doi.org/10.1002/ggge.20177.
Xu, G., et al. (2014), Time-series measurement of hydrothermal heat flux at the Grotto mound, Endeavour Segment, Juan de Fuca Ridge, Earth Planet. Sci. Lett., 404, 220–231, https://doi.org/10.1016/j.epsl.2014.07.040.
Xu, G., D. R. Jackson, and K. G. Bemis (2017), The relative effect of particles and turbulence on acoustic scattering from deep sea hydrothermal vent plumes revisited, J. Acoust. Soc. Am., 141, 1446–1458, https://doi.org/10.1121/1.4974828.
Author Information
Guangyu Xu (email: [email protected]), Woods Hole Oceanographic Institution, Mass.; Karen Bemis, Rutgers University, New Brunswick, N.J.; and Darrell Jackson, Applied Physics Laboratory, University of Washington, Seattle
Citation:
Xu, G.,Bemis, K., and Jackson, D. (2017), Sounding the black smoker plumes, Eos, 98, https://doi.org/10.1029/2017EO086289. Published on 10 November 2017.
Text © 2017. The authors. CC BY-NC-ND 3.0
Except where otherwise noted, images are subject to copyright. Any reuse without express permission from the copyright owner is prohibited.